At the Physics Department of HKUST, a group of researchers has been carrying out work on the superlensing effect in photonic crystals. We are:
- Che Ting Chan (theorist)
- Xinhua Hu (theorist)
The lensing effect can be achieved in photonic crystal (PC) slabs, but in many situations, the mechanism can be traced to the self-collimation effect of a square-like constant frequency surface. We show that using a metal-in-dielectric configuration, circular constant frequency surfaces can be obtained, and the lensing effect then obeys fairly well the image-distance relationship characteristic of an n = -1 material. As a two-dimensional example, far-field imaging is realized in square arrays of silver nanowires in air for transverse-electric waves at 400 nm. A high resolution of about 100 nm is obtained with a three-layer slab of such PCs. By varying the matrix, the results are extended to the optic and near-infrared regimes.
[1] Xinhua Hu and C. T. Chan, Appl. Phys. Lett. 85, 1520 (2004).
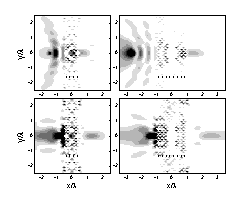